Water Treatment for Cathodic Electrodeposition Coating Processes
Cathodic electrodeposition coating is most commonly applied in the automotive industry to protect vehicle bodies and components against corrosion. In the automotive sector, it generally serves as the first layer of the primary painting process, acting as a primer that provides high adhesion and corrosion resistance on metal surfaces. Additionally, due to its ability to achieve uniform and complete coverage even on complex-shaped parts, this method has found extensive applications in various industries.
For example, cathodic electrodeposition coating is used in agricultural and construction machinery, truck and bus chassis and components, household appliances and durable consumer goods, electrical panels and switchgear equipment, metal office furniture, garden equipment, marine industry components, fasteners (such as bolts and nuts), and many other fields. In these industries, cathodic electrodeposition coating has become a crucial process to enhance the resistance of parts against atmospheric conditions (such as humidity and saltwater) and to provide long-lasting corrosion protection.
For instance, steel, aluminum, or magnesium parts coated with cathodic electrodeposition coating can withstand at least 1000 hours in salt spray tests and can provide an additional 6 to 12 years of rust resistance in real-world applications. Due to this superior protection, cathodic electrodeposition coating can be used alone, particularly in applications such as chassis and underbody protection, where "black cathodic electrodeposition" primer is commonly applied. However, it is often complemented with topcoats such as wet paint or powder coating, forming a complete coating system.
Key Water Treatment Systems for Cathodic Electrodeposition Coating
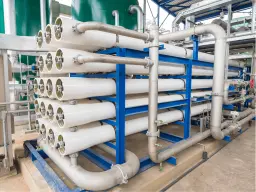
Reverse Osmosis
Uses semipermeable membranes to remove dissolved impurities, ensuring high-purity water for boiler feed.
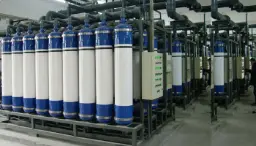
Ultrafiltration
Removes suspended solids and colloids as a pretreatment step, enhancing downstream performance.
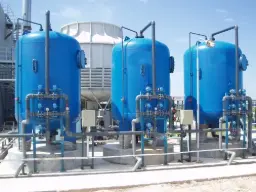
Water Softener
Exchanges calcium and magnesium with sodium, significantly reducing scale buildup in boilers.
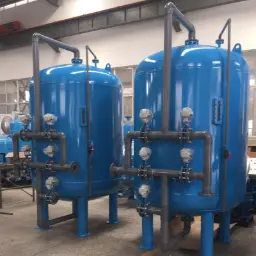
Deionization
Employs ion exchange resins to produce nearly pure water, minimizing damaging contaminants.
What is Cathodic Electrodeposition Coating?
Cathodic electrodeposition coating, also known as electrophoretic coating, is a surface protection method based on the principle of depositing paint particles on a conductive surface with the help of electric current (). This method allows resin and pigment particles suspended in a liquid paint bath to accumulate as a uniform film on the metal surface with the effect of the applied direct current. Although the first electrophoretic painting patent was received in 1917, its industrial applications began in the mid-20th century. Especially in the 1960s, anodic electro-coating (anophoresis) techniques began to be applied on small parts, but due to the anode being a piece, dissolution and corrosion problems were observed on the metal surface. Cathodic electro-coating technology (cataphoresis), which was developed to eliminate these problems, was first introduced as a commercial facility by PPG Industries (USA) in 1970 and was first used in the automotive industry in 1975 (). In 1978, the first car body in Europe was painted with cataphoresis primer (Chrysler plant in France) and the process has developed rapidly since then. Today, cataphoresis painting has become an environmentally clean (heavy metal-free), economical and superior corrosion protection technology. Modern cataphoresis paints have been developed to be more environmentally friendly (e.g. lead-free) and have low volatile organic compound (VOC) content than previous generations. In addition, the efficiency and performance of the coating process has increased continuously over the years; for example, automotive body anti-corrosion warranties, which were around 6 years in the 1980s, have now increased to 10-12 years.
Cathodic Electrodeposition Coating Process Steps
The cathodic electrodeposition coating process is generally considered in three main stages: (1) Surface preparation , (2) Electro-coating (cataphoresis) application , (3) Curing (baking) . The details and sub-steps of these stages are explained below.
Surface Preparation (Cleaning and Phosphating)
Before starting the cathodic electrodeposition coating process, the surface of the parts must be prepared appropriately. Surface preparation aims to remove residues such as oil, dirt, dust, rust and oxide layers that may be on the part and to make the surface suitable for coating. A typical surface preparation process includes multi-stage chemical baths:
Degreasing (alkaline cleaning): In the first step, the parts are cleaned with detergent/alkaline chemicals in one or more stages to remove the oil and dirt layer on them. This process can be done by spray and/or immersion method. For example, in an automotive cataphoresis line, both spray and immersion degreasing baths can be used consecutively.
Rinsing: After degreasing, the parts are rinsed with water to remove cleaning chemicals and dissolved dirt. Usually, the first rinse is done with city water, followed by a second rinse with deionized (DI) water.
Activation: Before phosphate coating, an activation bath is applied to promote the formation of a homogeneous and fine crystalline phosphate layer on the metal surface. Activation is usually carried out with a low concentration solution containing titanium or nickel and improves the nucleation of the phosphating reaction.
Phosphating (Conversion coating): A critical step in surface preparation, parts are usually immersed in a zinc phosphate or iron phosphate bath to form an insoluble phosphate conversion layer on the metal surface. Zinc phosphating is widely used in applications where high corrosion resistance is required, such as automotive, and leaves a fine crystalline zinc-phosphate layer on the surface. This layer provides a rough and reactive surface that significantly increases the adhesion and corrosion resistance of cataphoresis paint.
Passivation (Pause) and Rinses: After phosphating, the parts are rinsed with water again and a passivation step may be applied to remove any remaining soluble salts on the surface. Passivation provides additional protection against corrosion by treating the phosphate coating with a silicate or organic-based coating and increases the stability of the phosphate layer. Although chromate-based passivations have traditionally been used, chromium-free passivation chemicals are now generally preferred for environmental and health reasons. Finally, just before entering the cataphoresis bath, the parts are rinsed one last time with deionized water to ensure that no conductive residue or dirt remains on the surface.
Good surface preparation is essential for the success of cathodic electrodeposition coating. A clean and active surface ensures strong adhesion of the coating to the substrate and the formation of a homogeneous film. An improper pretreatment can lead to problems such as corrosion progression under the coating, paint blistering or poor adhesion after coating.
Cathodic Electrodeposition Coating Bath and Electrochemical Deposition
The pretreated clean and phosphated parts are immersed in a cathodic cataphoresis dye bath. In this bath, the parts are connected to a negative charge (cathode); the soluble anodes immersed in the tank are connected to a positive potential. The cataphoresis bath consists largely of pure water and dispersed dye solids: typically about 80-90% deionized water and 10-20% dye solids (resin + pigment). The deionized water serves as the carrier medium for the dye solids; the dye solids consist of the resin (binding polymer) and pigments that form the coating film . The resin is the main component in the final film, providing properties such as corrosion resistance, mechanical strength and chemical resistance; the pigments provide the film with properties such as color, opacity and UV resistance. The formulation of cataphoresis paints also contains very small amounts of organic solvents and additives (around 5%), which are used to adjust viscosity, improve film properties and stabilize the bath.
When the parts are immersed in the paint bath, an electrochemical reaction begins with the application of direct current. The positively charged resin-pigment particles directed towards the surface of the part in the cathode position move towards the part (electrophoresis) and precipitate with the discharge of charge on the surface. The applied voltage level is the main parameter determining the paint film thickness . Applying sufficient voltage allows the paint to penetrate and accumulate even to the most recessed corners; thus, every area of complex shaped parts, including inner surfaces, weld seams, corners and edges, can be coated. In typical cathodic cataphoresis applications, the bath voltage can be in the range of approximately 100-300 V; ~250 V application is common for large automotive bodies. The parts are kept under current in the bath for a few minutes (e.g. 2-4 minutes). Since there is a strong electrical attraction between the bare metal surface and the conductive paint dispersion at the beginning, the current density is high and a rapid coating is formed. As the coating film thickens, the film begins to form a dielectric barrier and the conductivity of the part surface decreases. Once the target thickness is reached, the film electrically insulates the part and the current flow naturally decreases, balancing the coating build-up . Thanks to this self-stopping mechanism, a coating of approximately equal thickness is achieved at every point and over-coating is prevented.
The precipitation of paint particles on the cathode is also closely related to the electrolysis of water. Hydroxyl (OH^–) ions are formed as a result of the dissociation of water at the cathode, and this local pH increase renders the resin, which has previously been neutralized by acid and dispersed in the water, insoluble. The resin and pigments adhere to the cathode surface at this point, forming a film. The reverse reaction occurs at the anode, where the acidic byproducts accumulated in the system are generally removed from the bath by the membrane cells (anolyte circuit) surrounding the anodes. The acidic permeate solution collected in the anode chamber is periodically drained or neutralized, thus maintaining the bath chemistry in balance and limiting the pH increase. This balancing is critical to maintaining the stability of the emulsion by maintaining the pH in the range of approximately 5.7–6.0 under continuous process conditions.
In the cataphoresis bath, water and other components are released along with the sedimentation of paint particles. Therefore, the conductivity and ionic density tend to increase over time in the operating bath. Bath conductivity is typically controlled in the range of ~1000-2000 µS/cm; if conductivity increases too much, coating thickness control may become difficult or side reactions may increase. Therefore, a certain amount of permeate solution is withdrawn from the bath using ultrafiltration (UF) units and purified outside the system, and clean pure water or deionized water is added to keep the conductivity within the desired range. For DI water treatment, reverse osmosis or electrodeionization systems can be use. In addition, concentrated dye (resin-pigment paste) and neutralizing agent are added to the dye bath at certain intervals to keep the chemical composition of the bath (solid matter percentage, degree of neutralization, etc.) constant.
Rinsing and Post-Coating Processes
After the coating process in the cataphoresis bath is completed, the parts are slowly removed from the bath and immediately enter the rinsing stations after coating. The purpose of this stage is to recover the excess paint that has overflowed from the bath on the part (the “cream coating” layer that has not yet adhered to the surface) and to increase the smoothness of the coating. The first rinse is usually performed with ultrafiltered permeate solution taken from the cataphoresis bath. The excess paint particles filtered from the part are returned to the system thanks to this rinse; the paint accumulated in the rinse tanks is concentrated by filtration and fed back to the main paint tank. Thanks to this recovery principle, the paint material efficiency in the process can exceed 95% . In other words, almost all the paint on the surface of the part coming out of the bath either remains on the part as a film or is returned to the tank with rinses, and the amount of paint going to waste is very low.
After the first rough rinse, a one or two-stage deionized water rinse is performed. At this stage, the aim is to ensure that no paint residue or conductive material remains on the parts. In particular, the final rinse is performed with completely pure water and the parts are sent directly to the oven without waiting for a short time before being baked. The coating on the surface of the parts in the form of a wet film must be protected from deterioration during the rinsing before baking; therefore, the transition from rinsing to the oven must be fast and the environment must be kept free from contaminants such as dust and dirt.
In some systems, after the rinsing stage, the parts may be briefly placed in a low-temperature “flash-off” chamber before going into the furnace. This allows some of the water to evaporate, helping to prevent defects that can occur in the furnace due to flash evaporation (e.g. boil-off bubbles). However, in most modern cataphoresis lines, wet parts coming directly from the rinse enter the furnace at a controlled speed, and most of the water has already evaporated during the heating process.
Baking and Curing
The parts coated with cataphoresis and rinsed are baked in an oven to chemically cure the paint and form a solid film. At this stage, the parts enter the drying/curing oven with a conveyor system. The oven temperature and time are determined by the curing properties of the paint material used. Typical cathodic epoxy cataphoresis paints are fully polymerized (cross-linked) by raising the part temperature to approximately 160-200°C and holding for 20-30 minutes. For example, in common applications, a minimum of 20 minutes of curing is required at a part temperature of 190°C . This is usually achieved by setting the oven air temperature to ~180-200°C and by sufficient conveyor speed according to the heat capacity of the part. While thin sheet materials heat up faster, thick cast parts may require longer periods to reach core temperature. Paint manufacturers' technical sheets specify the minimum time-temperature combination required for curing (e.g. equivalent curing conditions such as 20 min/180°C or 30 min/160°C).
During curing, the resin (usually epoxy resin) in the cataphoresis paint hardens through a chemical reaction. Under the influence of heat, the reactive groups (e.g. epoxy rings) in the resin open up and form cross-links, creating a thermally resistant, rigid polymer network structure. In this way, the coating film becomes a dense protective layer that adheres strongly to the substrate and is resistant to impact and scratches. In addition, during the baking process, volatile components in the paint film (residues of water and organic solvents) evaporate and disappear. Since modern cataphoresis paints have a low volatile content, gas waste in the oven is relatively low and is usually purified by thermal oxidation. At the end of curing, the parts are left to cool immediately after leaving the oven; cooling usually occurs in ambient air, but forced cooling with fans may also be applied in some lines. After the coated parts have cooled to the touch, they are transferred to the next production step (e.g. sanding and topcoat painting in automotive or directly to the assembly line).
Final Quality Control
The final stage of the cathodic electrodeposition coating process is the quality control of the coated parts. In this step, various tests and measurements are used to verify whether the coating meets the desired properties:
Visual Inspection: The parts are checked visually and according to standards for any uncoated areas (bare metal), color or tone differences, surface deposits or grains, leaks, bubbles, etc. Cathodic electrodeposition coating usually forms a semi-matte solid color (black or gray) primer layer; therefore, the coating is expected to appear homogeneous. Especially in parts with complex geometry, critical areas are checked against the risk of paint not reaching the inner parts (lack of throwpower). If necessary, the inner surface coating can be examined by taking sections. Edge-corner coating is also evaluated; for this purpose, some standard test pieces (e.g. sharp-edged samples) are coated in the process and the film thickness on the edges is examined.
Thickness Measurement: Coating film thickness is a critical parameter for performance and is measured on each batch. Measurement is usually done with non-destructive magnetic induction or eddy current based thickness measuring devices (e.g. hand-held devices for measuring paint thickness on steel, ISO 2178/ASTM D7091 compliant). Typical cataphoresis primer thickness is around 15-35 µm ; ~20 µm is targeted for automotive applications. Measured values should be within the desired tolerance range (e.g. \pm2-3 µm). Process settings are reviewed as under-thickness may lead to insufficient protection; over-thickness indicates economic loss and risk of paint cracking. Therefore, thickness values are constantly monitored.
Adhesion Test: The adhesion resistance of the coating to the substrate is evaluated by standard scratch-scratch or cross-cut tests. A common method is the cross-cut adhesion test ; in this test, a square pattern is drawn on the coating surface with a sharp knife in a controlled manner and an adhesive tape is attached and pulled. It is classified from 0 to 5 according to the ISO 2409 standard according to the remaining paint percentage. In cathodic electrodeposition coatings, a result of Gt0 or Gt1 (0–5% paint removal) is expected, which is considered the best class. For example, in a good cathodic electrodeposition coating, all cut edges should remain smooth in the cross-cut test and no square should peel completely.
Hardness and Strength Tests: Mechanical tests such as hardness (pencil hardness test, pendulum test) and impact resistance (falling weight test) can be applied to the coating film when necessary. Cataphoresis primers generally form a hard film (pencil hardness is usually around H-2H). In addition, stone impact resistance (gravel test) is a feature that is checked especially in automotive parts; it is desired that the coating does not crack or suffer minimum damage from gravel impact.
Corrosion Resistance Tests: The corrosion resistance of the coating is verified by accelerated aging tests at certain intervals or on a sample basis. The most common test is the salt spray test (salt fog test, 5% NaCl spray, 35°C ambient, ISO 9227 / ASTM B117 standard). Cataphoresis primers are usually formulated to withstand salt fog test for over 500 hours ; automotive requirements often require >1000 hours of rust-free resistance. At the end of the test, the presence of blistering or rust progression under the coating is measured according to ASTM or ISO assessor standards (e.g. rust progression distance in mm). For example, a system may target <1 mm in 504 hours of salt test and <1.5 mm in 1008 hours. In addition, humidity/condensation tests (ISO 6270-2, 240-480 hours at conditions such as continuous 95% humidity, 40°C) can be applied and then adhesion is checked. Climate cycling tests (variable temperature-humidity-salt cycles such as VDA 621-415) and thermal shock tests can also be performed depending on customer requirements. All these accelerated tests are used as an indication that the cathodic electrodeposition coating will provide corrosion resistance in real life for many years.
Other Checks: The electrical insulation of the coating is already high in proportion to its thickness; however, for special applications, dielectric strength measurements can be made. Chemical resistance tests (e.g. resistance to contact with fuel, engine oil, brake fluid, antifreeze) can be important for automotive parts and are tested according to standards. Colour or gloss are generally not critical for the primer (since the topcoat is applied over it), but in cataphoresis applications used for decorative purposes (e.g. chassis parts with a single coat of black coating), colour uniformity and UV resistance can be checked. Since epoxy cataphoresis paints can chalk in UV light, the need for a polyurethane-based top coat for parts to be used in direct sunlight is also taken into account in quality planning.
Parameters to be Measured and Controlled
In order to ensure quality and continuity in the cathodic electrodeposition coating process, both the chemical parameters of the bath conditions and the electrical/physical parameters of the application are regularly monitored. In addition, some measurements are made at the exit to ensure that the coating obtained meets certain quality criteria. In this section, the critical parameters and their typical value ranges are discussed:
Chemical Bath Parameters: The chemical balance of the cataphoresis paint bath directly affects the coating quality. First of all, the pH value is a critical parameter. In cathodic systems, the paint bath pH is typically kept in the slightly acidic region, approximately 5.7 - 6.0 . This range provides sufficient neutralization for the resin to remain water-soluble (emulsified), but since it does not rise too high, the resin has not yet precipitated. If the pH value goes beyond this optimum range, undesirable results will occur: For example, if the pH increases, the solubility of the resin decreases, emulsion stability deteriorates, and coagulation may begin in the paint tank. This leads to clogging of filters and membranes, and the bath may become muddy. Conversely, if the pH drops too low (too acidic), this time acidic corrosion increases in the installation, and since metal ions such as iron can dissolve, contamination of the paint color and membrane clogging may occur. Therefore, the pH is measured daily and adjusted with chemical additions when necessary.
Another important parameter is the conductivity (Conductivity = electrical conductivity, usually in µS/cm). Bath conductivity indicates the ability of the paint to carry current by conduction and affects the current density during coating. In an operating cataphoresis bath, conductivity tends to increase over time due to by-products formed during coating (soluble salts), added neutralizers (e.g. acid or amine) and conductivity contributions from concentrated paint. If conductivity increases too much, it indicates that undesirable conductive impurities have accumulated in the bath and may affect the evenness of the coating. Typically, cataphoresis bath conductivity is controlled in the range of 800 – 2500 µS/cm (note: although some sources express this in mS, in practice it is in the range of ~1-2.5 mS/cm). If the upper limit of conductivity is exceeded, it is usually reduced by removing some of the bath solution by ultrafiltration and adding pure water. Reverse osmosis or DI water treatmen system are required for this process. In addition, increasing the solvent level or changing the bath temperature are among the methods to adjust conductivity.
Bath temperature is controlled because it affects chemical and electrokinetic parameters. High temperatures can make the paint dispersion unstable or increase evaporation, while low temperatures can reduce coating speed and current efficiency. Most cataphoresis baths are operated at or slightly above room temperature (e.g. 28-32°C). Temperature also affects viscosity, which is inversely proportional to conductivity; increasing the temperature can make the paint fluid and increase conductivity somewhat. For this reason, tanks are equipped with heating/cooling coils and the temperature is kept constant.
Solid content (dye concentration): The percentage of solid paint in the bath should generally be within a certain range. A typical cathodic primer bath is expected to contain 12-15% solid content (for example, 14-18% is desired depending on the formulation). This value affects the coating thickness and efficiency; if it is too low, it becomes difficult to achieve the desired film thickness, if it is too high, the bath viscosity increases and its stability may be impaired. The amount of solid content is measured by the method of drying and weighing bath samples taken at certain intervals using gravimetric analysis . For example, 1-2 g of bath sample is dried in an oven at 110°C and the residue percentage is calculated.
Acid value (Total/Neutralizing Acid): Cataphoresis paints are generally neutralized with organic acids to make the resin water-soluble (in cathodic systems, a positively charged amino-resin is formed with the organic acid neutralizer). As the bath ages, the amounts of free and bound acids may change. Therefore, the total acid number and free acid values are monitored with titration analyses. For example, the acidity number is calculated by adding suitable solvents to a certain volume of bath sample and titrating with 0.1 N NaOH with a pH meter. Paint manufacturers usually give a target range for the “acid ratio” (e.g. acid ratio), which indicates the degree of neutralization of the resin and therefore its ability to load onto the part. This parameter is controlled and neutralizer (amine) or acid is added to the bath when necessary.
In addition to the above, other chemical parameters such as the density of the bath , viscosity , particle size distribution of the pigments dispersed in it, and the amount of sludge/sediment can also be monitored. However, it can be said that the most critical ones routinely are pH, conductivity, solid percentage and acidity. Keeping these parameters within the desired ranges is ensured by regular analyses every day/week and proactive measures are taken by following the trends.
Electrochemical Parameters: Since the cataphoresis process is an electro-coating process, the electrical control parameters are also very important. The applied voltage is the most basic parameter; typically, a direct current power unit (rectifier) is set to the desired voltage value and this voltage is maintained throughout the coating process. Depending on the product type, values between 50-400 V can be used. A higher voltage allows more paint particles to be drawn rapidly, resulting in a thicker film; however, very high voltages can trigger undesirable electrolysis side effects (e.g. hydrogen gas bubbles on the metal surface, pin-hole defects). Therefore, there is an experimentally determined optimum voltage profile for each product and paint. For example, in automotive body cataphoresis, the initial coating time is rapidly increased to 250 V, held constant for a while, and then reduced according to the current drop. The current density varies depending on the geometry and surface area of the parts; a high current (e.g. a few amperes/dm²) is usually seen at the beginning of the coating and decreases as the film forms. The total load (ampere-minute) passed in the process is related to the coating thickness. For this reason, ampere-minute control is also performed in advanced systems: A target A min value is calculated for each tank according to the part surface area and the desired coating thickness, and the coating is terminated when this value is reached. Electrical parameters are usually monitored continuously via the automation system (PLC); instantaneous voltage, current and time values are recorded. If there is a deviation from the desired range (for example, if the current is too low, there may be an electrical contact problem with the part hanging, if it is too high, there may be a bath conductivity/pH problem), the operator is immediately alarmed and corrected.
Coating Quality and Homogeneity: The process parameters must be correct so that the coating forms a uniform film at every point. As stated in quality control, homogeneous distribution of the coating is important. To ensure this, throw power and edge-corner coating performance are taken into account during the process. For example, immersion time and voltage are optimized to ensure sufficient coating in deep pockets. Some productions use standard test panels to measure this performance. In the Ford automotive industry's throw power test, the percentage of coating that reaches the inner surface of a certain geometric panel is measured (e.g. 60-65% throw distance). For edge protection, coating thickness can be evaluated on sharp-edged samples. Mixing/circulation systems also affect homogeneity in the process; continuous low-speed mixers or circulation pumps in the bath ensure that the paint particles are constantly suspended and present in equal concentration in each region. If mixing is insufficient, regional concentration differences in the tank can cause fluctuations in the coating. Therefore, in order to ensure homogeneity of both bath conditions and electrical distribution, correct pumping, anode arrangement and hanger (hanger apparatus) design are made in the plant design. The way the parts are hung on the hanger is also important: Air outlet from the pocket areas on the part and bath drainage should be good so that the paint can enter everywhere and not cause pooling at the exit.
Film Thickness and Corrosion Resistance: These two properties are the most critical performance indicators desired in the final product. Although film thickness is measured and controlled during production (see the quality control section), it is also related to the process parameters. For example, higher thickness can be achieved by increasing the voltage or time. However, there is a certain economic and technical optimum for cataphoresis (usually ~20 µm); thicker films are generally not recommended because they waste paint material and can cause problems with the top coats. Corrosion resistance is the main performance criterion of a correctly applied cathodic electrodeposition coating. As mentioned above, it is measured by standard tests and must be above a certain threshold. Process parameters affecting corrosion resistance include surface preparation (quality of phosphate), film thickness, degree of curing, and paint formulation. Therefore, these parameters are controlled throughout the process to ensure that the targeted corrosion resistance level (for example, the maximum rust progression criterion determined in the 500-hour salt test for an automotive part) is achieved in each batch.
Quality and Process Standards: Cathodic electrodeposition coating process and results are also defined by international standards. For example, ISO and ASTM standards that provide general test methods for paint coatings are also valid for cataphoresis. Process control plans of cataphoresis lines are created and recorded within the scope of quality management standards such as ISO 9001 and especially IATF 16949 for automotive . Technically, adhesion control is performed with ISO 2409 (cross-cut adhesion test) and Gt0 or Gt1 is generally expected. ISO 2808 defines paint dry film thickness measurement methods and thickness measurements of cathodic electrodeposition coatings can be made according to this standard. ISO 6270-2 is the humidity cabinet test standard and measures the resistance of cataphoresis coated samples in wet hot environment (e.g. there should be no deterioration in adhesion after 240 or 480 hours) (). ISO 9227 (equivalent to old DIN 50021 or EN ISO 7253) is the salt spray test standard and is used to evaluate the corrosion resistance of cataphoresis primer. In addition, automotive manufacturers have their own cathodic electrodeposition coating specifications (for example, many OEM companies have material specification numbers [BMW, Ford, VW, etc.] as listed by Ege Eloksal). For the environmental aspect of the process, values are checked in terms of EPA standards or local environmental legislation in terms of waste water and volatile emissions; for example, in Europe, heavy metals (lead, mercury, cadmium, Cr6+) are not used in cataphoresis paints due to the ELV directive. In short, the cathodic electrodeposition coating process is carried out in accordance with national and international standards and the products are also verified according to the relevant test standards.
Measurement and Control Methods
The control of the above-mentioned parameters in the cathodic electrodeposition coating process is carried out with various measurement methods and devices. Regular measurements ensure that the process remains stable and possible deviations can be detected and corrected early. In addition, quality control tests are carried out with established methods. This section describes the measurement techniques of important parameters, how the results are interpreted and process improvement approaches:
Bath Chemical Analysis: A series of chemical analyses are performed on samples taken daily or weekly from the cataphoresis bath. pH measurement is performed with a digital pH meter. The sample temperature is brought to a standard value (usually 25°C) and the measurement is recorded with a calibrated glass electrode pH meter. For example, most facilities measure pH at least once a day and check that the ideal range of ~5.7-6.0 is maintained. In case of pH deviation, the operator adds corrective chemicals according to the instructions of the paint supplier (such as a controlled acid dose to the system if pH has increased, or a neutralizing amine if pH has decreased).
Conductivity measurement is done with a calibrated conductivity meter. The bath sample is measured with a certain cell constant probe and the conductivity value is read in µS/cm. This value is usually desired to remain in a moderate range (e.g. 1000-1500 µS/cm). Facilities also record conductivity daily and follow the trend. If conductivity tends to increase continuously, this is an indication of bath accumulation; as a solution, a small amount of bath discharge can be made and pure water can be added or UF waste output can be increased. For example, in some facilities, if the conductivity exceeds a certain threshold value (~2000 µS), an alarm is given and ultrafiltration is automatically activated to remove excess conductive ions. Many facilities, uses PLC systems for control and feed baths with pure water from pure water treatment systems when increase the conductivity.
Determination of solids (solid content) is a simple but critical test. For this purpose, a small aluminum container is weighed and a certain amount (1-2 g) of bath sample is placed in it and dried in an oven at 105-110°C until it reaches a constant weight. The amount of dry film remaining after drying is weighed and the percentage is calculated. The result is expected to be around 15% of the sample (target range is defined by the manufacturer). This test is performed at least once a week. If the solids are low, it means that the concentration of the dye has decreased; generally, some fresh dye (resin/pigment concentrate) is added from the dye feed system. If the solids are high, there is excess dye accumulation in the system; the addition of pure water or reduction of dye feed is considered.
Total acid and free acid analysis is done by titration method. Laboratory technician dilutes a certain volume of bath sample with deionized water and suitable organic solvent (e.g. isopropanol or THF) and titrates with 0.1 N NaOH solution in the presence of phenolphthalein indicator under magnetic stirring. At the end point (around pH ~8.5) acidity number (mg KOH/g or similar unit) is calculated from the consumed NaOH volume. This value is an indirect measure of the amount of neutralizer in the paint. For example, a target such as “total acid value = 40 mg KOH/g” can be. In addition, free acid (unbound acid) is determined with a second titration and the ratio of the two (free/total) is evaluated. If the titration results do not comply with the specification, neutralization balance is adjusted by adding amine or acid to the bath.
Bath temperature and other environmental parameters are also measured, but these are usually monitored continuously by sensors in the automation system. However, operators will read tank thermometers or review data records for cross-checking. If the temperature is different from the desired temperature, heaters or coolers will be adjusted. The amount of sludge/cementation in the bath is also monitored; if necessary, the bath will be clarified at regular intervals or the filtration systems will be maintained.
Electroplating Process Controls: Electrical parameters used during coating are monitored and controlled digitally in modern facilities. Values such as voltage, current and time can be viewed instantly from the rectifier screens or the SCADA software interface. Operators verify that the correct program is selected and that voltage ramps are appropriate at the beginning of each batch. For example, a slow voltage increase profile may be required for large parts, while a fast increase may be used for small parts. The current time curve is also monitored; if the current drops to zero much earlier than expected, the film may be insulating quickly (perhaps excessively thick or the bath solids are high), or if the current does not drop, the coating may not be complete (perhaps poor surface preparation or low conductivity). The operator gets an idea about the process according to these signs. If necessary, test panels are added to the rack and the current distribution during coating is evaluated.
In addition, issues such as the conductivity of the hanger apparatus, the condition of the anodes, and gas release during the coating process are monitored by the workshop personnel. Regular cleaning is performed to prevent paint accumulation and insulation on the hangers. Anode membranes are periodically checked, and clogged ones are cleaned or renewed (if the anolyte circuit does not work properly, the bath pH may go out of control). All these control activities are aimed at ensuring that the process parameters remain within defined tolerance ranges .
Quality Test Methods: Tests performed on the final coating are applied with certain standard methods (what these are mentioned above). For example, we said that a magnetic thickness gauge is used for thickness measurement ; these devices generally work according to ISO 2178 or ASTM D1186. In order for the device to give accurate results, it must be calibrated with various calibration foils. The operator tries the device on test plates with known thicknesses before measurement. During the measurement, values are taken from several different points on each piece and the average is recorded. If the cross-cut adhesion test is performed according to ISO 2409, it is important to use a standard-sized sharp knife and tape; the results are graded according to the classes defined in the standard () (). A salt spray cabin is used in accordance with the ISO 9227 method for the salt test ; the test can be performed at certain periods (e.g. by taking a painted sample from each production lot) or the samples are tested for periods such as 240, 480, 720 hours for product approval. At the end of the test, the degree of rust is evaluated according to ASTM D1654 or ISO 4628. Humidity tests are performed in a constant temperature/humidity cabinet in accordance with ISO 6270-2; at the end of the period, the sample is checked for swelling and the change is checked with the adhesion test (). Impact test can be performed with the device according to ASTM D2794. Hardness can be measured with ISO 1518 (pencil hardness) or ISO 1522 (pendulum hardness König/Persoz), etc. The methods and acceptance criteria of all these tests are specified in the quality plans and are implemented by trained personnel.
Interpretation of results is based on relevant standards and customer requirements. If any test result is out of specification, corrective measures are taken on the products of that batch (e.g. a second cataphoresis or additional preservative treatment in consultation with the customer) or production is stopped and process settings are reviewed. For example, if a poor adhesion test is performed, surface preparation tanks and paint bath chemical values are checked immediately; usually the problem lies in either the phosphate layer or an imbalance in the paint bath.
Process Improvement Methods: Keeping the cathodic electrodeposition coating process under control as well as developing it with a continuous improvement approach is common in the industry. In this context, the measurement data obtained can be analyzed with statistical process control techniques in the long term. For example, the process capacity can be evaluated by monitoring the fluctuations of critical parameters such as pH and conductivity with SPC cards; If the Cpk values are low, it may be necessary to increase the chemical feeding automation or to perform more frequent analysis for improvement. Similarly, potential causes and precautions for coating failure are determined by performing a failure mode and effects analysis (FMEA).
Some of the common applications for process improvement are: Optimizing the paint material (for example, saving energy by using new generation cataphoresis paints that can be cured at lower temperatures, energy recovery (heat recovery of hot air from ovens with heat exchangers), increasing automation (transferring manual controls to automatic sensors and PID controls), better hanger design (jig designs that improve paint deposition and current distribution on the part), regular maintenance and cleaning (preventing contamination by cleaning anodes, filters, and tanks), training (continuous training of operators on the effects of process variables), and chemical optimization (for example, reducing sludge waste by using nano-ceramic pretreatment instead of phosphate).
Also, if a facility requires cataphoresis with different colors (which is rare), strategic decisions can be made, such as setting up two separate lines, as color changes in a single bath are difficult – this also relates to the flexibility of the process. Process planning is also part of the improvement (e.g. using a single primer color and applying color in top coats approach), as cataphoresis is not suitable for situations where frequent color changes are required.
In summary, measurement and control methods should be used not only to monitor the current situation, but also to see trends and make continuous improvements. Even small improvements in the cataphoresis process (e.g. increasing the bath life from 6 to 12 months, reducing energy consumption by 5%, reducing the defective part rate by 1 per thousand) can provide great economic and environmental benefits. Therefore, industry and academia are conducting various studies in cooperation to make this process more efficient, more environmentally friendly and higher performing.
Resources and Academic Studies
There is a wealth of literature on cathodic electrodeposition coating, both academic research and industry-focused technical documents. This process has been developed and documented over the years with many scientific studies, especially since it plays a critical role in the automotive industry. Some important sources and studies on the subject are summarized below:
Academic Research: Universities and research organizations have published studies on the corrosion performance of cathodic electrodeposition coating, its interaction with materials, and process parameters. For example, Akafuah and colleagues (2016) published a comprehensive review article examining the evolution of the automotive body painting process, detailing the historical development and modern improvements of cataphoresis technology () (). This study addressed issues such as increased corrosion resistance, the shift to environmentally friendly formulations (e.g. lead-free paints), and energy efficiency in the transition from first-generation anodic systems to today's high-performance cathodic systems. Again, at the academic level, a study by Yargıç et al. (2021) examined the performance of hot forged casting materials under different coating conditions and evaluated the contribution of cathodic electrodeposition coating to the corrosion and wear resistance of these materials. Kılınç and Akyalçın (2022) investigated how corrosion performance was improved with duplex coating (e.g. zinc flake coating and top coat paints) applied to cataphoresis-coated steel surfaces . Such studies provide guidance for optimum protection of cathodic electrodeposition coating alone or in combined systems. In fact, according to a statistic reported by Kılınç (2019), thousands of patents have been obtained worldwide regarding cathodic electrodeposition coating; this is an indication of continuous innovation and development activity ().
Master's and Doctoral Theses: There are also academic theses on the subject in Turkey. For example, Özçanak (2008) has conducted a comprehensive study titled “Investigation of Surface Preparation, Drying and Coating Processes Before Cathodic Electrodeposition Coating” in his master's thesis at Yıldız Technical University . In this thesis, the effect of pretreatments on cataphoresis quality was experimentally investigated and the results of different phosphating and drying conditions were evaluated. Such theses are valuable in terms of analyzing real processes in the industry in a laboratory environment and making suggestions for improvement. Similarly, theses have been conducted on topics such as paint chemistry, coating adhesion, and analysis of coating defects related to cataphoresis at various universities.
Industry Reports and Technical Documentation: Automotive major industries and paint manufacturers publish technical reports and guides on the cataphoresis process. For example, major paint companies such as PPG, Axalta, and BASF provide cathodic electrodeposition coating technical sheets and guide documents. A technical document from PPG describes the steps of the cataphoresis process (pretreatment, coating, rinsing, and oven) in detail; it is stated that the bath composition is 80-90% water and 10-20% solids , that the coating yield exceeds 95%, and that typical cure conditions are 190°C for 20 minutes . Such documents are a reference source for process engineers and technicians. Again, automotive companies' material specifications are important industry references; for example, manufacturers such as Ford, GM, Volkswagen, and Toyota have developed standards detailing the tests and properties that cataphoresis primers must meet. These specifications define a number of criteria such as coating thickness, salt test resistance hours, stone impact test results, and electrical insulation. Suppliers of cathodic electrodeposition coating prove that they meet these criteria with test reports that comply with these OEM standards.
Energy and Environmental Studies: Energy consumption and environmental impacts during the operation of cataphoresis facilities are also the subject of research. For example, Akbaş et al. (2018) considered the cataphoresis line as a separate process while performing the energy consumption analysis of an automobile assembly plant and revealed where energy savings could be made, especially in baking. This study includes guiding data for the industry on heat optimization of cataphoresis furnaces, recovery systems and general line efficiency. On the environmental side, there are technical reports on wastewater treatment (waste from phosphating and painting tanks) and the reduction of volatile organic compound emissions. For example, in the BREF (Best Available Techniques Reference) documents published in the European Union, best practices and emission control techniques, including cataphoresis, are defined for surface coating facilities.
Standards and Guides: International standards organizations (ISO, ASTM, DIN, TS etc.) have published some standards directly related to cataphoresis. For example, ISO 10683 and EN 13858 , although more related to zinc-lamellar coatings, also cover the requirements for the use of electro-coatings such as cataphoresis on fasteners (). In addition, ASTM has standard test methods specific to electrophoretic coatings (such as ASTM B767 – electro-coating thickness measurement). These standards are also used as references in academic studies.
As a result, there is a wide range of theoretical and practical knowledge about the cathodic electrodeposition coating process. Scientific research deepens the understanding of the process, while industry-specific documents provide guidance for application. The sources cited in this report and other similar literature are important references that reveal the development of cathodic electrodeposition coating, optimal application conditions and quality control. It is anticipated that in future studies, environmentally friendly material development (e.g. new generation chrome-free pretreatment, resins that can be cured at lower temperatures) and process optimization (e.g. artificial intelligence-supported bath control) will be at the forefront. This continuous development will ensure that cathodic electrodeposition coating continues to occupy a critical place in various industries.